Molecule of the Month: Directed Evolution of Enzymes
Biological evolution is being harnessed in the lab to create new enzymes.
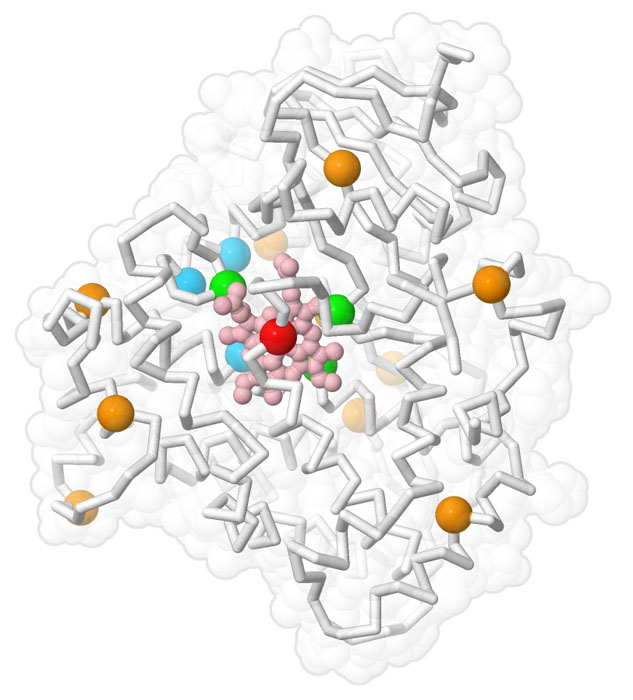
Directed Evolution
Tricky Chemistry
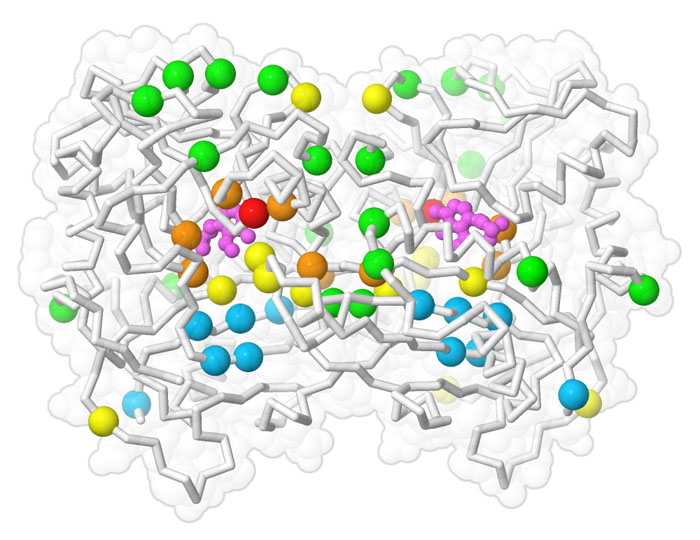
Building Drugs
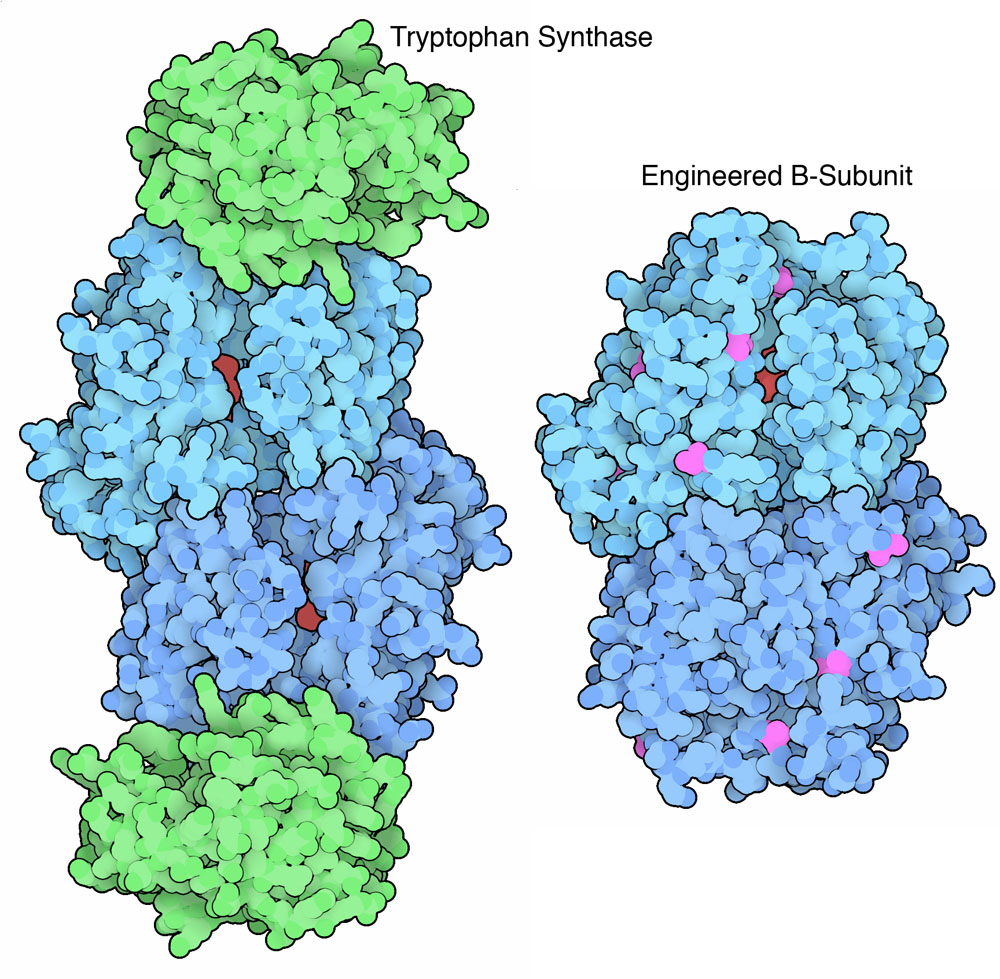
Extending Nature
Exploring the Structure
Cofactor Binding to an Evolved Enzyme
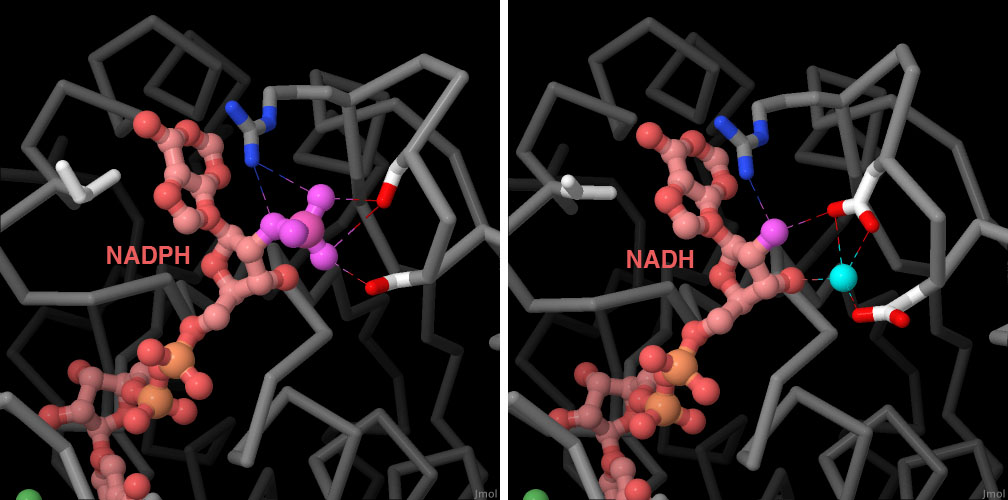
Directed evolution has also been used to tune biochemical pathways in cells. For example, the Arnold lab modified the pathway for production of isobutanol, a second-generation biofuel. Two of the enzymes in the isobutanol production pathway, alcohol dehydrogenase and KARI, use the cofactor NADPH. However, the similar cofactor NADH is much more prevalent in these cells, since they generate much of their energy through glycolysis. So, Arnold and her team used directed evolution to modify the enzymes so that they can use NADH instead of NADPH. For alcohol dehydrogenase, variants that use NADH are found in other organisms, so one was engineered for optimal function in this pathway, but for KARI, the entire enzyme needed to be evolved to switch its preference from NADPH to NADH. A similar cofactor shift was engineered into the related KARI enzyme shown here, with the original enzyme on the left (PDB entry 4kqw) and an evolved NADH-dependent enzyme on the right (PDB entry 4kqx). Click on the image to explore these enzymes in more detail.
Topics for Further Discussion
- Many other examples of directed evolution, both for enzymes and for other proteins, are available in the archive. Try searching for “directed evolution” to see some of them.
- See other examples of molecular evolution in the new Browser topic on Molecular Evolution.
Related PDB-101 Resources
- Browse Nobel Prizes and PDB structures
- Browse Renewable Energy
- Browse Biotechnology
- Browse Molecular Evolution
References
- Arnold, F.H. Directed evolution: bringing new chemistry to life (2018) Angewandte Chemie International Edition 57: 4143-4148.
- 6am8: Buller, A.R., van Roye, P., Cahn, J.K.B., Scheele, R.A., Herger, M., Arnold, F.H. Directed evolution mimics allosteric activation by stepwise tuning of the conformational ensemble (2018) Journal of the American Chemical Society 140: 7256-7266.
- 5ucw: Prier, C.K., Zhang, R.K., Buller, A.R., Brinkmann-Chen, S., Arnold, F.H. Enantioselective, intermolecular benzylic C–H amination catalysed by an engineered iron-haem enzyme. (2017) Nature Chemistry 9: 629-634.
- 3wwj: Guan, L.J., Ohtsuka, J., Okai, M., Miyakawa, T., Mase, T., Zhi, Y., Hou, F., Ito, N., Iwasaki, A., Yasohara, Y., Tanokura, M. A new target region for changing the substrate specificity of amine transaminases (2015) Scientific Reports 5: 10753.
- 4kqw, 4kqx: Brinkmann-Chen, S., Flock, T., Cahn, J.K., Snow, C.D., Brustad, E.M., McIntosh, J.A., Meinhold, P., Zhang, L., Arnold, F.H. General approach to reversing ketol-acid reductoisomerase cofactor dependence from NADPH to NADH (2013) Proceedings of the National Academy of Science USA 110: 10946-10951.
- Savile, C.K., Janey, J.M., Mundorff, E.C., Moore, J.C., Tam, S., Jarvis, W.R., Colbeck, J.C., Krebber, A., Fleitz, F.J., Brands, J., Devine, P.N., Huisman, G.W., Hughes, G.J. Biocatalytic asymmetric synthesis of chiral amines from ketones applied to sitagliptin manufacture (2010) Science 329: 305-309.
- 1wdw: Lee, S.J., Ogasahara, K., Ma, J., Nishio, K., Ishida, M., Yamagata, Y., Tsukihara, T., Yutani, K. Conformational changes in the tryptophan synthase from a hyperthermophile upon alpha(2)beta(2) complex formation: crystal structure of the complex (2005) Biochemistry 44: 11417-11427.
December 2018, David Goodsell
http://doi.org/10.2210/rcsb_pdb/mom_2018_12