Exploring the Structural Biology of Health and Nutrition
By understanding the molecular needs of our cells, we can ensure that our bodies operate at peak performance.
Our cells make about 20,000 types of proteins, as well as many types of nucleic acids, carbohydrates, lipids, and small molecules. By understanding the structure and function of these molecules, we can ensure that they are performing at their peak. This includes ensuring that we have sufficient raw materials to build and power all of these molecules, and knowing when we need to step in ourselves and modify the action of these molecules with drugs and other medical interventions. This knowledge informs the ways that we all can live our best lives, at all stages of our lives.
TOPICS
A balanced diet provides building blocks and energy for all our biomolecular machines
Vitamins are essential molecules that our cells cannot make themselves
Iron, calcium, and other minerals assist the action of proteins and are used to build healthy bones
Our cells and our bodies rapidly adjust to changes in the environment
A proper balance of cholesterol and other lipids is needed to build healthy membranes
Athletes can use understanding of molecular biology to enhance their abilities
Genetic disorders are often caused by life-threatening malfunctions of our molecules
Our molecules, with a few exceptions, have defined lifespans
1. A balanced diet provides building blocks and energy for all our biomolecular machines.
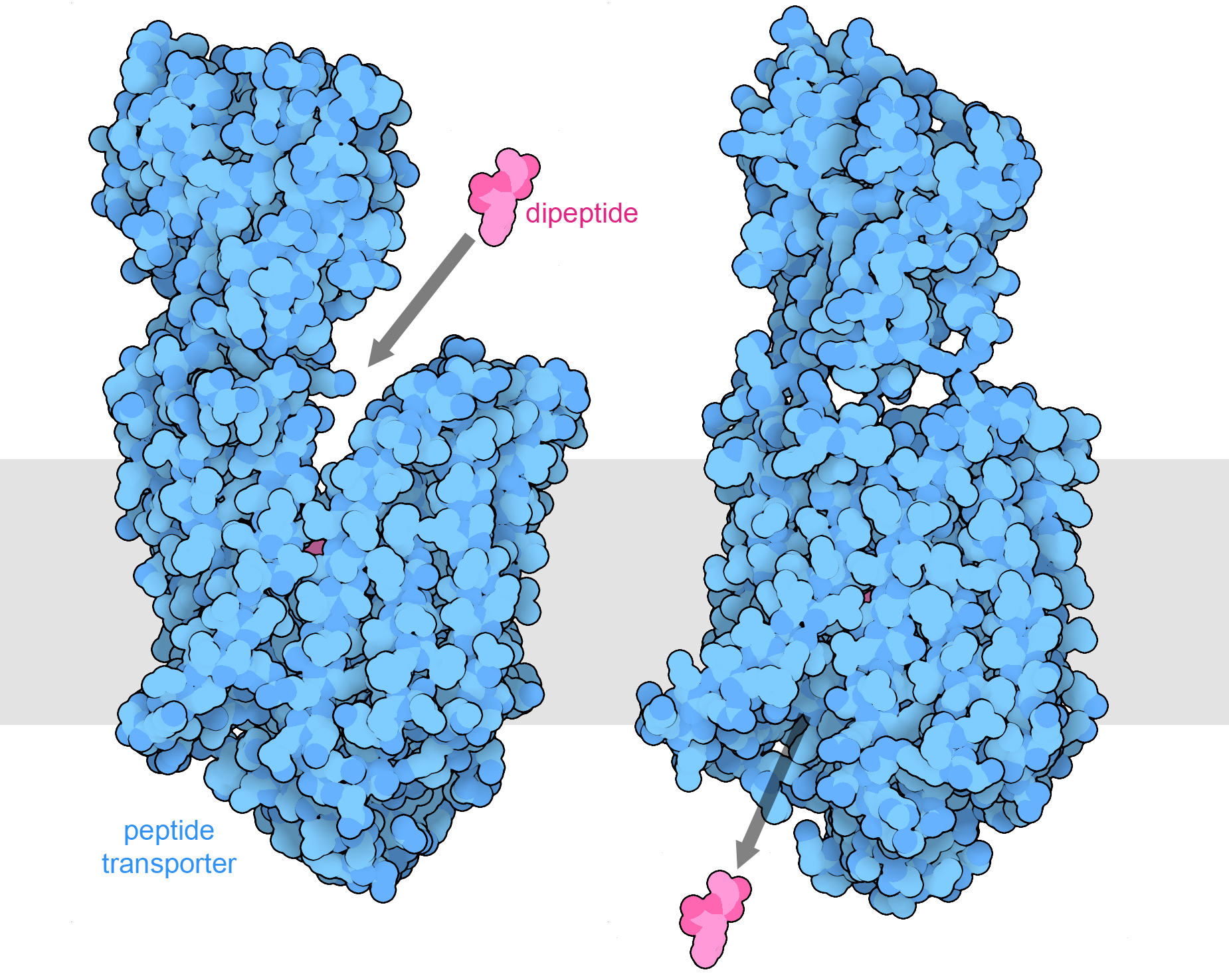
Peptide transporter 1 transports small peptides into intestinal cells. The structure on the left is open towards the outside of the cell and captures peptides, such as the alanine-phenylalanine dipeptide included in the structure (PDB ID 7pmx). The transporter also binds a hydrogen ion (not shown), flipping to a conformation that is open towards the interior of the cell, as seen in the structure of a similar transporter shown at right (PDB ID 7pmy). The location of the intestinal cell membrane is shown schematically in gray.
The food that we eat contains four major classes of molecules–proteins, nucleic acids, carbohydrates and lipids–as well as many micronutrients like vitamins and minerals. As seen in the many structures in the PDB archive, proteins are the most diverse class of large biological molecules, performing many different tasks. These include enzymes responsible for catalyzing specific chemical reactions, proteins involved in cellular signaling, and huge proteins like collagen that form the structure of our bodies. Nucleic acids largely focus on tasks related to genetic information, storing information in DNA and using it to build proteins with messenger and transfer RNAs and ribosomes. Carbohydrates play two major roles. First, small sugars like glucose are used for energy by pathways such as glycolysis and larger carbohydrates like glycogen and starch are used to store energy for later use. Second, carbohydrates play structural roles, for example forming a protective coat on glycoproteins on the exterior surface of our cells. Lipids form large sheets that surround our cells, as described in more detail in section 5 below.
When we eat these molecules, they are broken into smaller pieces by digestive enzymes such as pepsin, trypsin, amylases, and phospholipases. Then, the pieces are transported into the cells lining the digestive system by specialized transporters. For example, the one shown here captures small peptides that are two or three amino acids in length from the intestinal soup. Some of these building blocks are used to build new proteins and other molecules in our cells, and others are broken down to generate ATP and other forms of energy. Some of the molecules that we eat, such as the cellulose that surrounds plant cells, are not digestible and form the fiber that assists with normal movement of foodstuffs and waste products through our digestive system.
2. Vitamins are essential molecules that our cells cannot make themselves.
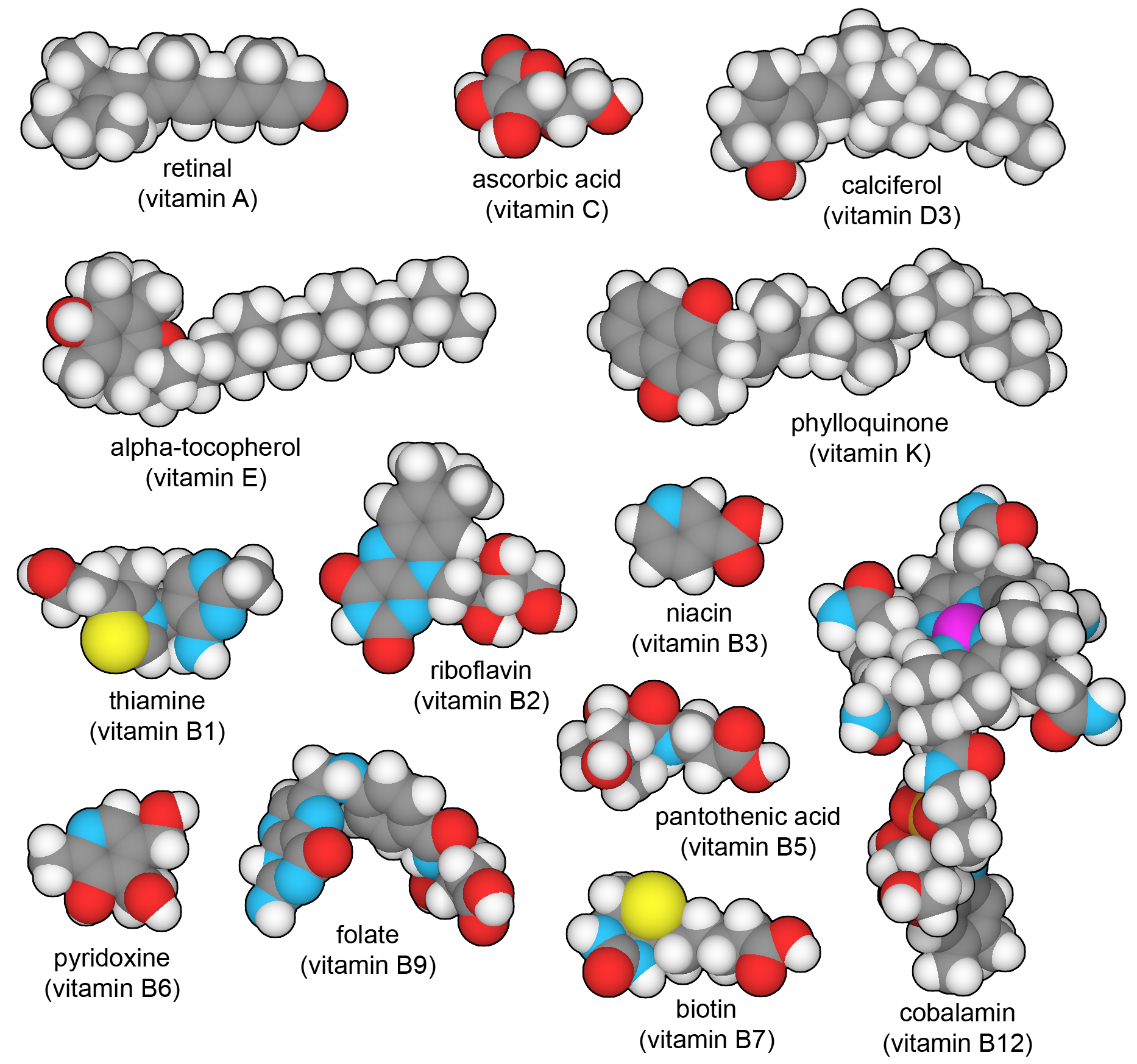
Thirteen vitamins that are essential in the human diet. You can explore these structures in PDB ligand IDs RET (A), VIB (B1), RBF (B2), NIO (B3), PAU (B5), UEG (B6), BTN (B7), FOL (B9), B12 (B12), ASC (C), VD3 (D), VIV (E), and PQN (K).
Our cells are filled with enzymes that build hundreds of different types of small molecules, including amino acids, nucleotides, sugars, cofactors, and many others. However, there are a few essential molecules that we cannot make ourselves, and must instead obtain pre-made in our diet. These include nine "essential" amino acids, which are abundant in a balanced diet. In addition, we require thirteen vitamin molecules that perform specialized tasks throughout the body. Vitamin A senses light in our eyes, B vitamins are experts at carrying chemical groups or single electrons from place to place, vitamins C and E are powerful antioxidants, vitamin D is an essential hormone for managing calcium levels, and vitamin K assists with the synthesis of special modified amino acids found in blood clotting factors. A balanced diet typically includes all of these exotic molecules, but if not, they will need to be obtained in vitamin supplements.
3. Iron, calcium, and other minerals assist the action of proteins and are used to build healthy bones.
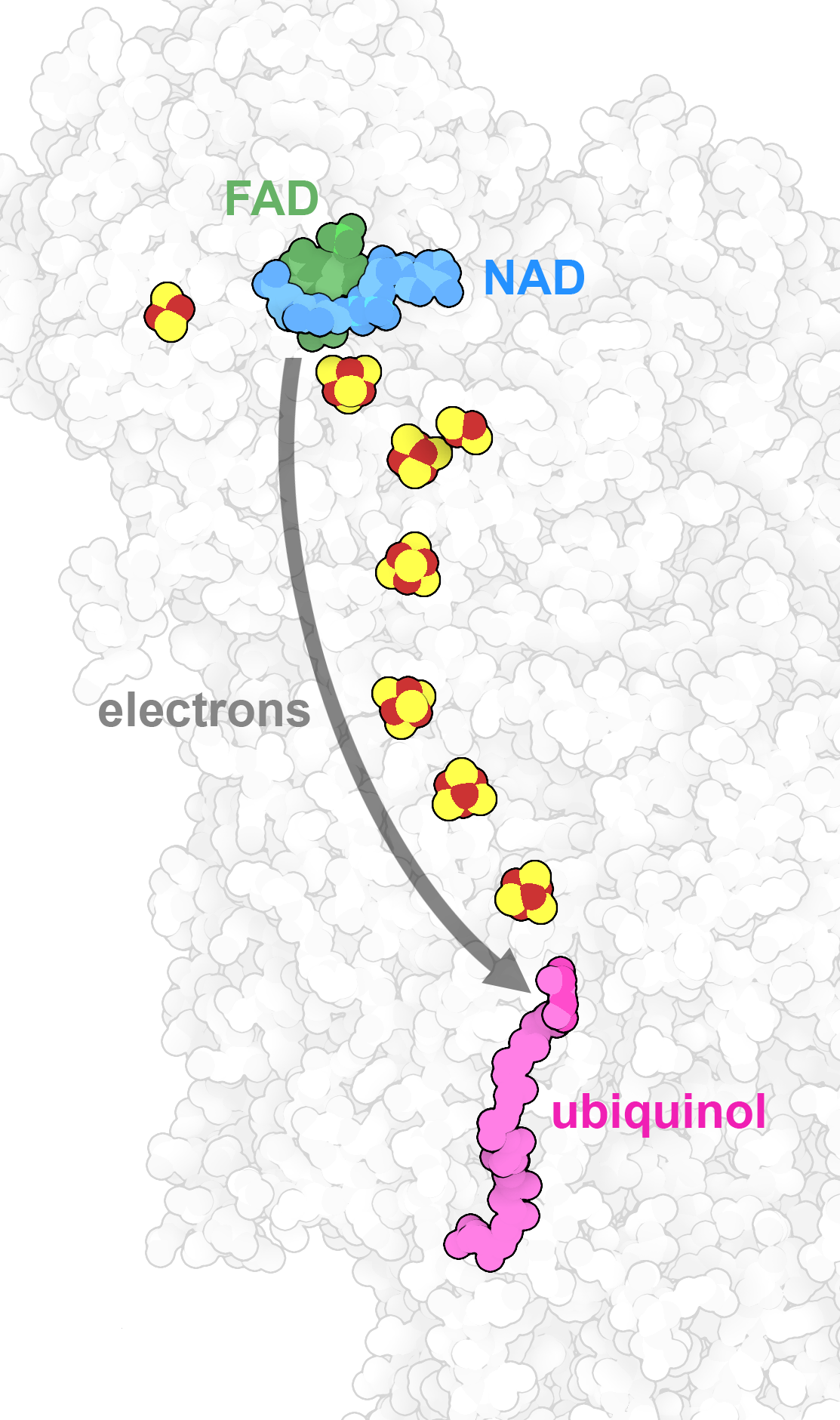
Inside NADH dehydrogenase, part of the respiratory supercomplex, a string of small iron-sulfur clusters form a molecular wire that transmits electrons from the carrier molecule NAD to ubiquinol. PDB IDs 7v2e and 7v2c.
For some tasks, organic molecules like proteins and nucleic acids aren't sufficient. Instead, our cells employ inorganic compounds with specialized properties. For example, dense crystals of calcium and phosphate provide the rock-like strength of our bones. Metal ions are used by many enzymes in several ways. Some proteins use them to hold particularly slippery molecules. For example, myoglobin and hemoglobin use iron atoms to capture individual molecules of oxygen, so that they can be stored within muscle or delivered through the bloodstream. Metal ions are also experts in managing single electrons. For example, the respiratory supercomplex uses long strings of iron and copper ions to extract electrons from the carrier NAD and place them on oxygen. Overall, we need small amounts of 15 or so different types of inorganic elements in our diet, including halogens like chlorine and iodine, selenium, and many types of metal ions.
Metal ions are also used to manage signaling over long distances. They are well suited to this task because they carry an electrical charge, and thus are easy to recognize, and they diffuse rapidly in the water environment of cells and tissues. Typically, a cell readies itself by pumping the ions across the cell membrane, using proteins such as the sodium/potassium pump and calcium pump to build a difference in concentration. Then, to send the signal, selective ion channels such as voltage-gated sodium channels and the calcium channel are opened allowing the ions to flow back. By arranging these pumps and channels appropriately, nerves send signals along their long axons and muscle cells coordinate their contraction. Since these pumps and channels are so important to the normal functioning of our nervous system, we have to be careful with the food that we eat. Many poisonous plants and animals protect themselves with powerful toxins that block these pumps and channels, such as digitalis toxins in foxglove, which blocks the sodium/potassium pump.
4. Our cells and our bodies rapidly adjust to changes in the environment.

The peptide hormone angiotensin helps regulate blood pressure. It is synthesized as a large precursor, angiotensinogen, which is cleaved first by the blood enzyme renin and then by ACE (angiotensin-converting enzyme). Finally, the short angiotensin II peptide binds to receptors on blood vessel cells, causing them to constrict and increase blood pressure. PDB IDs 3vcm, 2x0b, 1n9u, and 6os0.
The world around us is constantly changing, and we need to respond to those changes to stay alive and remain healthy. Our cells are constantly watching their local conditions and making changes in response. For example, most cells need a steady supply of glucose to power internal chemical processes, but glucose is only abundant right after we eat. So, we have a complex system that stores glucose as glycogen and releases it on demand, and a body-wide signaling system using insulin and glucagon to decide when to store glucose and when to release it.
TRPV1 channels watch for dangerous levels of heat and launch an appropriate response. Dangerously low levels of oxygen stimulate production of hypoxia-inducible factors, which tell cells to start using energy-production methods that don't need as much oxygen. By understanding these molecules, we can step in if things aren't going well. For example, problems with the glucose-signaling system lead to diabetes, which may be treated by administering insulin or other drugs at appropriate times. The signaling system shown here controls blood pressure, and several widely-used medications are used to block the angiotensin-converting enzyme to lower blood pressure that is too high.
5. A proper balance of cholesterol and other lipids is needed to build healthy membranes.
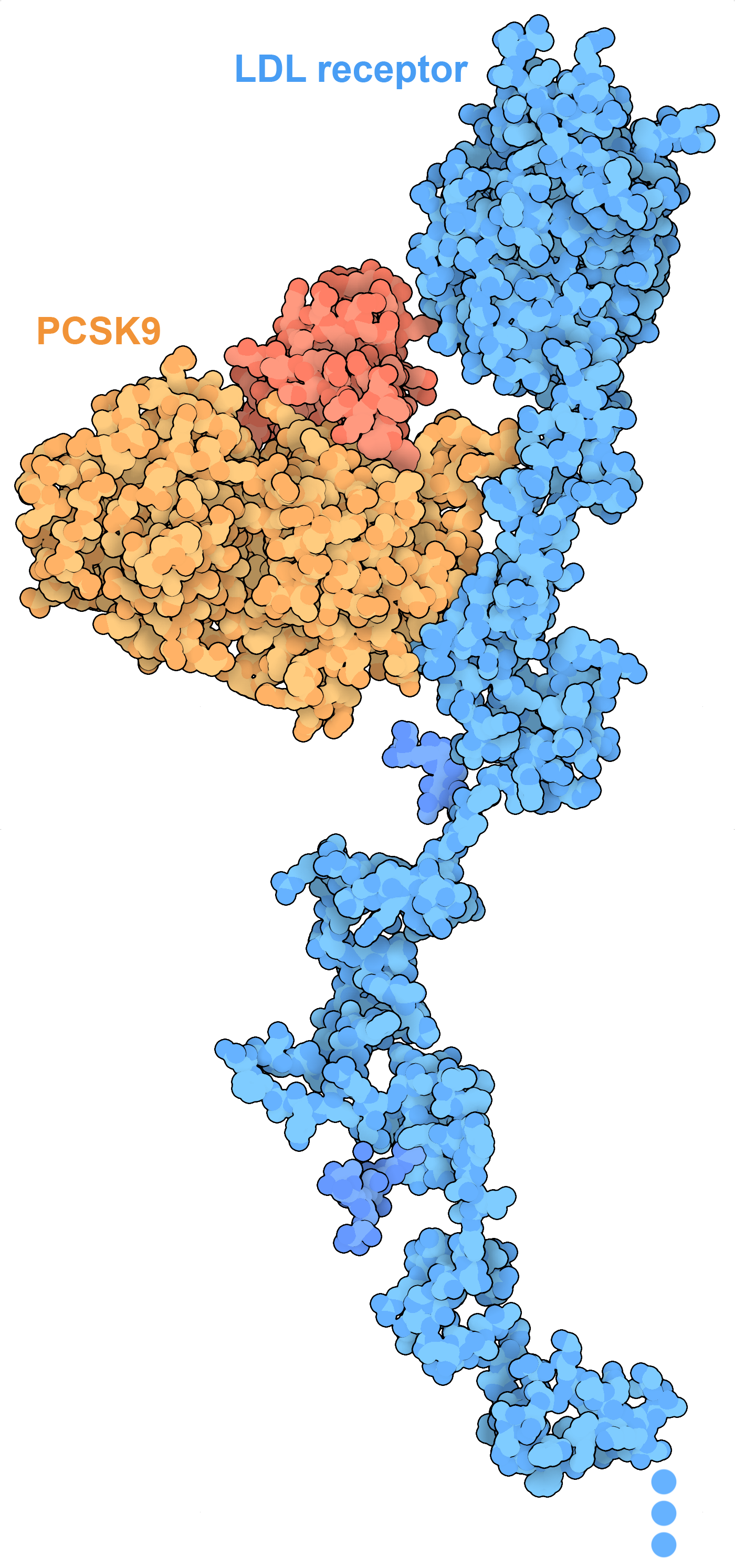
The extracellular portion of the LDL receptor (blue) binds to low density lipoproteins in the blood and transports them into the cell. PCSK9 manages degradation of unneeded LDL receptors. PDB IDs 3p5c and 1n7d.
Each of our cells is surrounded by a membrane composed of lipids, and similar membranes enclose functional compartments inside cells such as mitochondria and the nucleus. Lipids are perfectly structured molecules for this task. They are small molecules that associate into large, flexible sheets that can be used to build sealed, self-healing containers of many shapes and sizes. The properties of membranes can be easily tuned by inserting different types of lipids. Cellular membranes are also filled with proteins that span the membrane, transporting materials from one side to the other. Because membranes are random aggregates of lipids, they are difficult to study at the atomic level, and very few structures of them are available in the PDB archive. However, small pieces of membranes captured inside nanodiscs are being increasingly used to study membrane-bound proteins and several structures are available for exploration.
In spite of their essential functions in cells, lipids (and fats) have gotten a bad reputation when it comes to human health. Lipids and fats have a very high carbon content, which provide more energy when they are broken down than either carbohydrates and proteins. Thus, they are often used as a way to store energy when resources are plentiful. Proteins like leptin help our bodies control this by linking hunger with need for fat storage. Cholesterol has a dark side when it is too plentiful: when it is carried through the blood in low density lipoproteins (LDL), it can form deposits inside blood vessels that can lead to heart disease. By understanding the mechanisms of cholesterol synthesis and transport, therapies have been developed to keep cholesterol at optimal levels. For example, drugs such as atorvastatin are prescribed to block the enzyme HMG-CoA reductase, slowing the synthesis of cholesterol, and new treatments are targeting PCSK9 (shown here), a protein that controls the number of LDL receptors on the cell surface.
6. Athletes can use understanding of molecular biology to enhance their abilities.
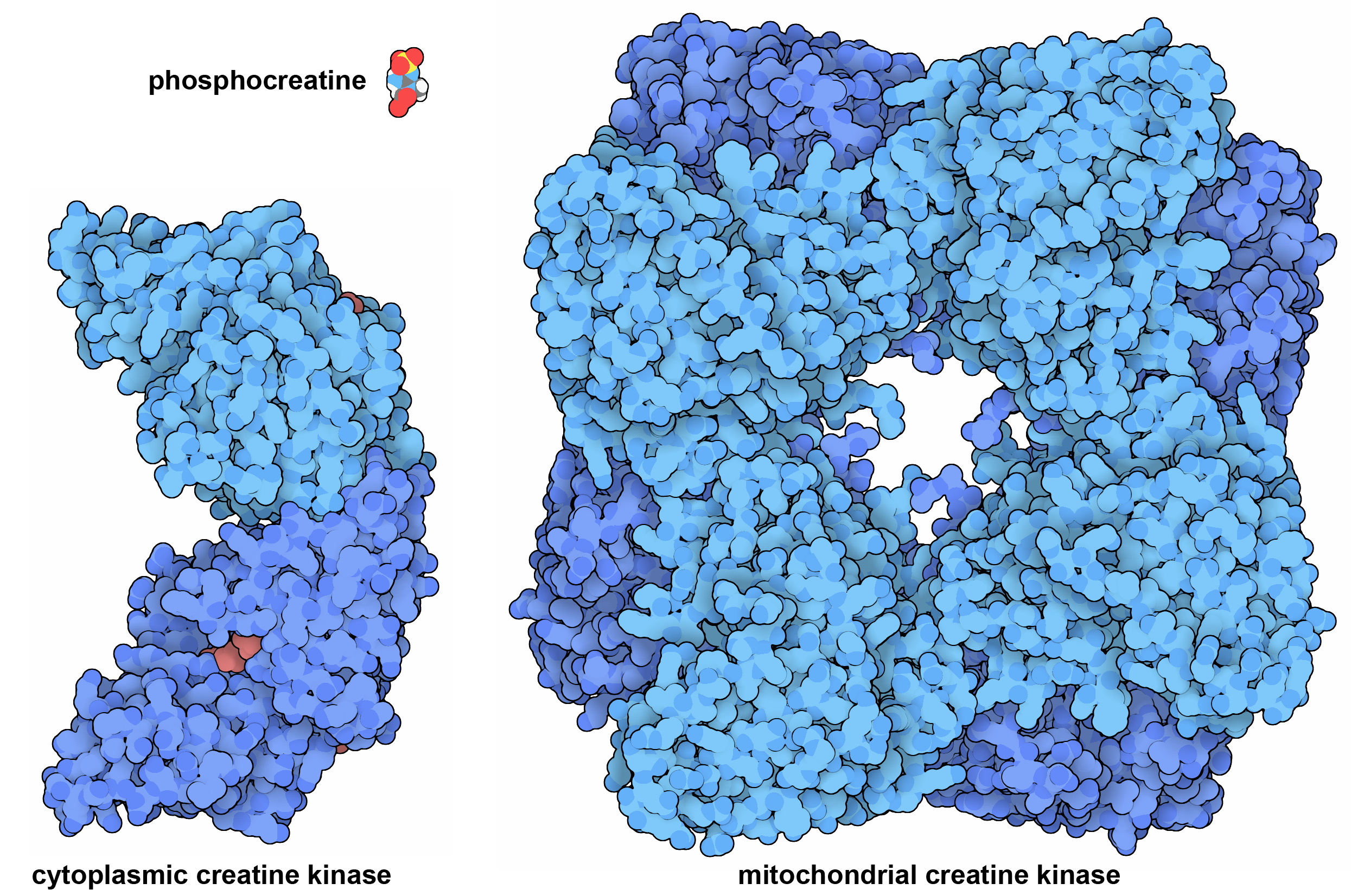
Our cells have two forms of creatine kinase, which together act as a shuttle to transfer energy. Mitochondrial creatine kinase builds a storehouse of phosphocreatine using ATP, and when needed, it is transported to the cytoplasm where cytoplasmic creatine kinase can use the phosphocreatine to build ATP. This system is primarily used in muscle cells, which have high energy demands to support their rapid contraction. PDB IDs 2crk and 1qk1.
Athletes are the embodiment of peak performance, showing the physical limits of what the human body can do. Knowledge of the biology of our bodies, from molecules to muscles, provides insights for how to improve this performance. For example, the common practice of "carb-loading" has its roots in the basic molecular biology of glucose utilization. Endurance athletes such as marathon runners need a lot of glucose to power their muscles, so they eat a lot of carbohydrates (and reduce their exercising) in the days before a big event. In that way, their muscles and liver build up a store of glycogen, and during the race, will provide a steady source of glucose. Basic biochemistry is also the basis of the adage "Slow and steady wins the race." Normally, our cells get plenty of oxygen from the blood and use a complicated pathway of aerobic respiration (glycolysis, citric acid cycle, and oxidative phosphorylation) to generate energy from glucose. However, if we exert energy too quickly, our cells can't get enough oxygen to support the normal pathways of energy production. These overworked cells rely on glycolysis for their energy, building up lactic acid as a waste product.
Athletes are always looking for ways to improve performance. Creatine supplements are widely used, for example. In cells, the small molecule creatine is used to store energy. It is phosphorylated by the enzyme creatine kinase, and phosphocreatine may later be used to build ATP with the reverse reaction. Creatine supplements have shown to provide some gains in sports performance and muscle growth. Other methods take a more invasive approach, and have largely been deemed unsportsmanlike for professional athletes. For example, one method of "blood doping" uses blood transfusions, filled with extra red blood cells and hemoglobin, to increase the transport of oxygen to working muscles. Anabolic steroids also have a checkered history in professional sports. They act as hormones that stimulate the body to build muscle, and are largely banned in events such as the Olympics and competitive team sports.
7. Genetic disorders are often caused by life-threatening malfunctions of our molecules.
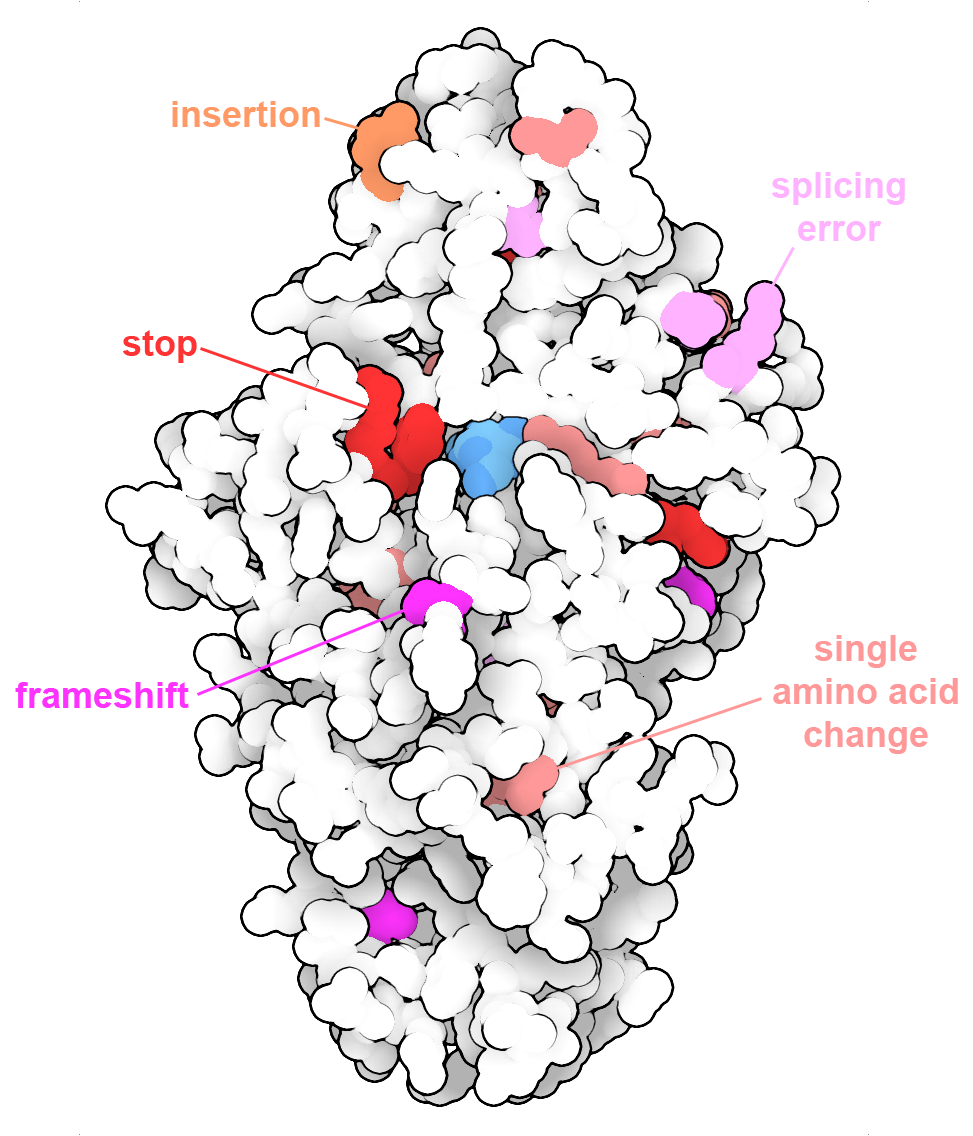
Tay-Sachs disease can be the result of many different changes in the gene encoding the enzyme beta-hexosaminidase. The alpha subunit is shown here with disease-causing changes, including modifications of single amino acids, insertion of extra amino acids or stop codons, and sites that cause frameshifts or splicing errors. PDB ID 2gk1.
Small changes in our genome can have huge effects on our health and welfare. For this reason, our DNA replication machinery is very accurate, with effective proofreading mechanisms, and we have excellent DNA repair mechanisms to correct the many errors caused by environmental dangers such as UV radiation. Errors do occur, however, and if they are present in the DNA contained in egg or sperm cells, they will be passed to children and on through subsequent generations. Many genetic disorders are caused by these types of small changes in a single gene, which can lead to synthesis of a faulty version of the protein that is encoded by the altered gene. For example, sickle cell anemia is caused by change of a single glutamate to a valine in hemoglobin, which makes the protein prone to aggregation into long filaments. Similarly, the weakened blood clotting in hemophilia is often caused by problems in blood clotting factors, which monitor the blood and initiate a clot when damage is sensed. Tay-Sachs disease is associated with mutations of the gene for beta-hexoaminidase, leading to faulty management of lipids and ultimately results in destruction of nerve cells.
Medical science is always looking for ways to treat these problems, but it is often difficult since it requires restoring the function of a faulty protein. Gene therapy is one option which is still under intensive study. In other cases, a drug may be found, for example, the drug ivacaftor enhances the function of some faulty forms of the cystic fibrosis transmembrane conductance regulator.
8. Many drugs selectively modify the action of our molecules.
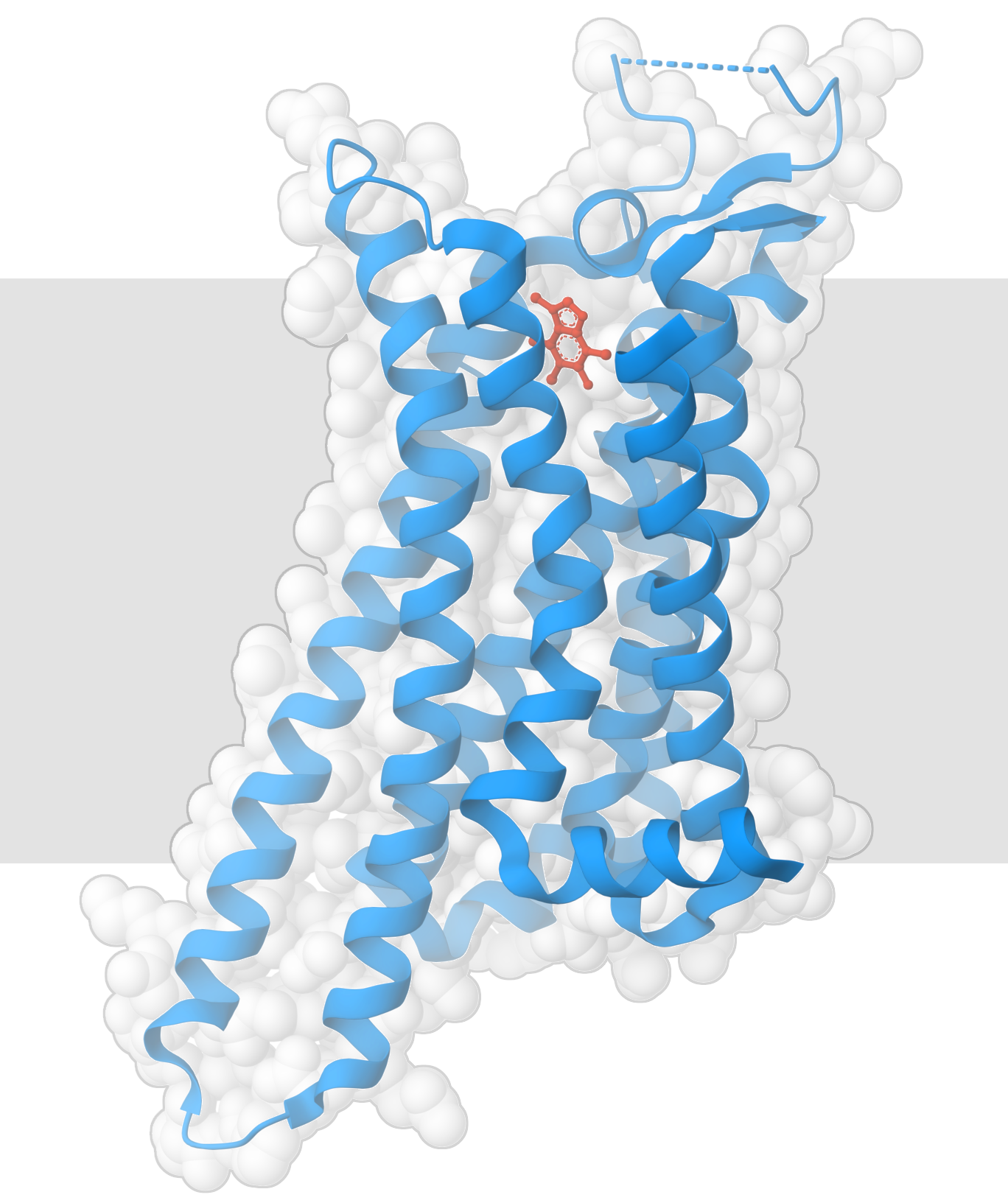
Caffeine binds to adenosine receptors (PDB ID 3rfm).
Amazingly, biological science gives us the ability to manage our own molecular machinery as needed. Drug molecules diffuse through the body and bind to target molecules, blocking or modifying their action. The most effective drugs are highly selective and only interact with the target of choice. Other drugs are not as selective, which may lead to unwanted side effects as they block the action of other molecules. Structures of target molecules allow us to understand how these drugs work, and to improve their binding strength and selectivity.
For example, if you drink coffee or tea, you're targeting adenosine receptors in your brain that manage wakefulness. When you take an aspirin (or ibuprofen or acetaminophen), you're blocking cyclooxygenase, an enzyme that creates molecules that carry pain signals. Other drugs can be life-savers. For example, beta-blocker drugs act on the adrenergic receptor and are used to manage abnormal heart rhythms. In keeping with the examples in this short list, many drugs target molecules involved in cellular signaling, which together manage the overall physiology of our bodies. In particular, the class of G-protein-coupled receptors (GPCR) are the targets of approximately half of all pharmaceuticals on the market today.
As seen in the current crisis of opioid addiction, some drugs come with a price. Opioids are highly effective for killing pain, but they are also strongly addictive. They act by blocking opioid receptors that manage our normal responses to pain.
9. Our molecules, with a few exceptions, have defined lifespans.
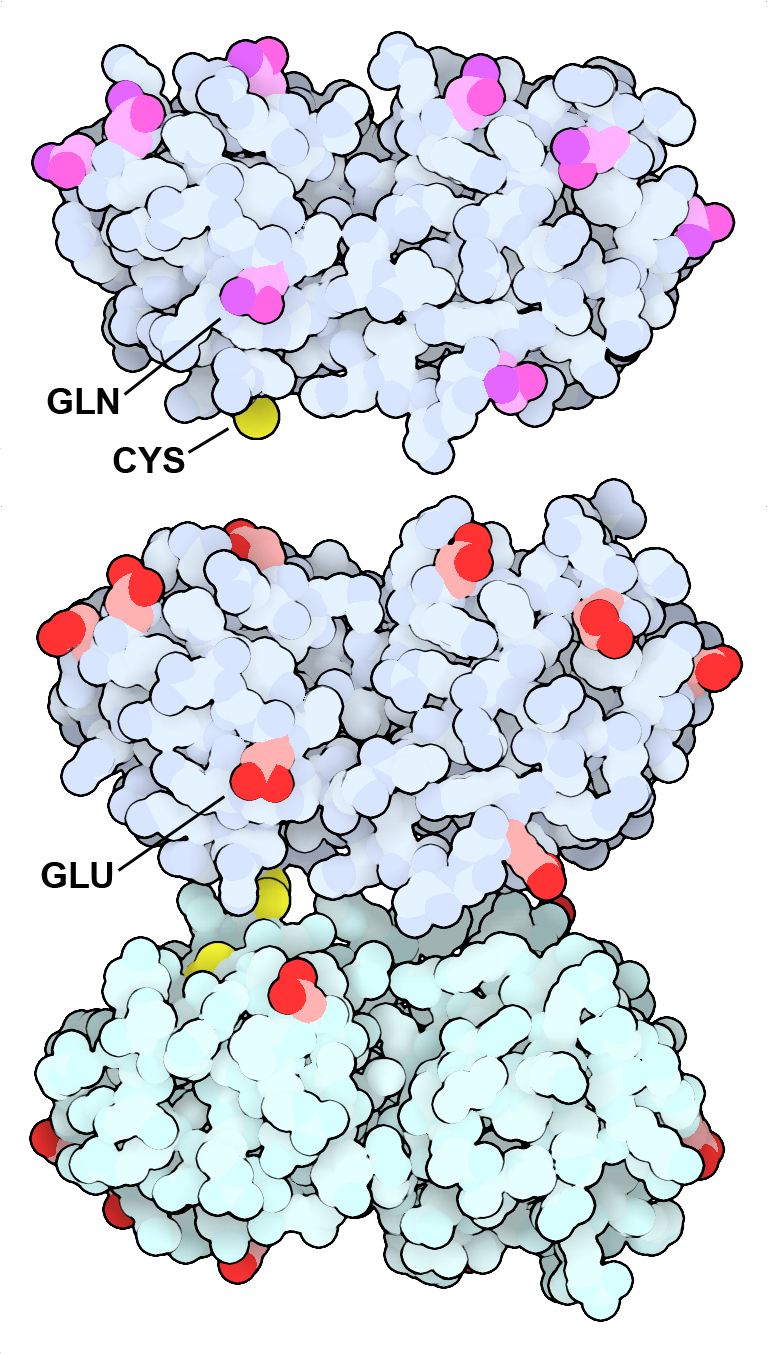
Gamma-S crystallin (top) has many glutamine and asparagine amino acids (magenta) on its surface. As the protein ages, many of these amino acids are deamidated to form acidic glutamate and aspartate amino acids (bottom, red), which promote the formation of a cystine-linked dimer. This form is also more prone to forming large aggregates, which scatter light and contribute to the opacity of cataracts. PDB IDs 7n36 and 7nje.
We have powerful mechanisms for recycling our molecules when they become obsolete. For example, most proteins in our cells have a life span of minutes to a few days. A short lifespan allows cells to tune their collection of proteins to respond to minute-by-minute changes in their environment. In addition, proteins become progressively damaged, for instance with deamidated or glycated amino acids, and need to be replaced. Our cells contain a powerful system for recognizing and tagging obsolete proteins with ubiquitin, then recycling them using proteasomes. In that way, our cells maintain a healthy "proteostasis," with only the proper types and amounts of each protein. Similarly, exosomes degrade obsolete messenger RNA molecules, ensuring that each cell is making only the most relevant new proteins.
There are, however, a few particularly long-lived proteins that last our entire lives. As you might expect, these are found in places in our bodies that are difficult to reach and repair. Examples include the crystallins in our eye lenses, dentin and enamel proteins in our teeth, and collagens and elastins in our connective tissue.
For more information, see:
Triggs-Raine, B, Mahuran, D. J., Gravel, R. A. (2001) Naturally occurring mutations in GM2 gangliosidosis: A compendium. Adv. Genet. 44, 199-224.
World Health Organization and Food and Agriculture Organization of the United Nations (2004) Vitamin and mineral requirements in human health, second edition. ISBN 9241546123
Toyama, B. H., Hetzer, M. W. (2013) Protein homeostasis: Live long, won't prosper. Nat. Rev. Mol. Cell Biol. 14, 55-61.
Wu, G. H., Chen, K. L., Hsu, C., Chen, H. C., Chen, J. Y., Yu, S. Y., Shiu, Y. J. (2022) Creatine supplementation for muscle growth: A scoping review of randomized clinical trials from 2012 to 2021. Nutrients 14, 1255.