Molecule of the Month: Glycolytic Enzymes
The ten enzymes of glycolysis break down sugar in our diet
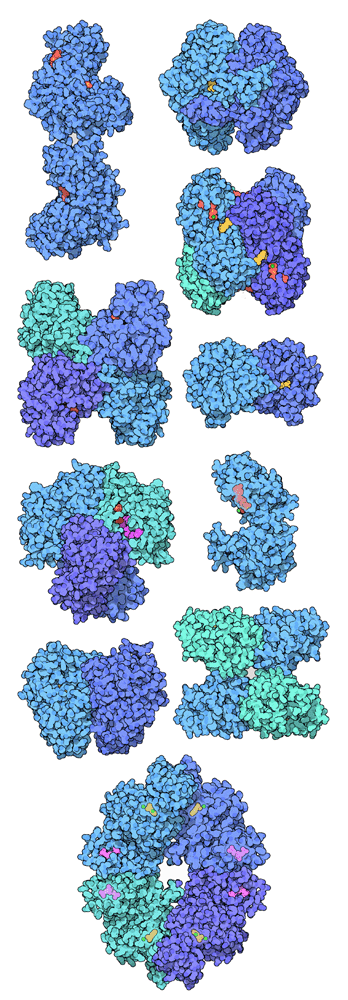
Sugar Breaking
After Glycolysis
A Perfect Ten
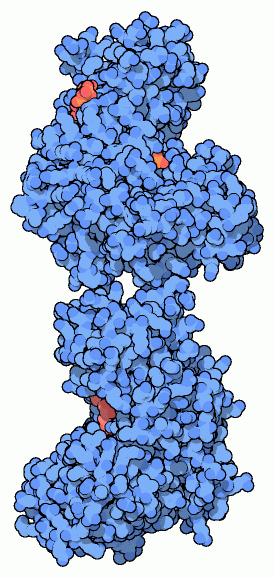
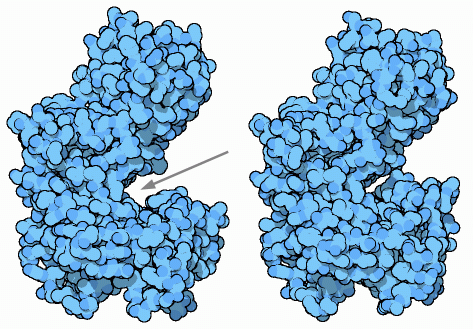
Hexokinase
As is often the case, when you look at the human form, things get more complicated. We build several types of hexokinase, made for the slightly different needs of different types of cells. The one shown here is from brain cells, from PDB entry 1dgk . It is twice as big as yeast hexokinase, and is amazingly constructed like two yeast enzymes strung together head-to-tail. Both halves have a nearly identical active site. But, the lower one has specialized in the catalytic reaction, while the upper one has specialized in regulation and does not perform the phosphate-transfer reaction.
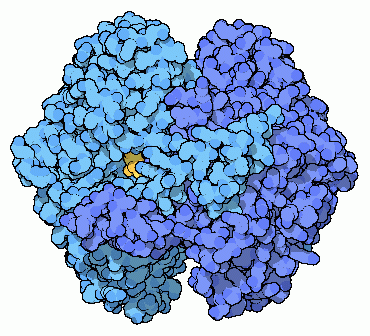
Phosphoglucose Isomerase
Recently, researchers have discovered that this protein also plays other important roles outside of cells, acting not as an enzyme but rather as a molecular messenger. It is secreted by white blood cells and helps control the growth and motion of many types of cells. As researchers delve deeper and deeper into the human genome, they are discovering numerous examples of other "moonlighting" proteins that have one function in one place in the body and an entirely different function somewhere else.
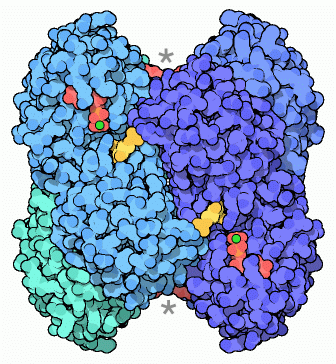
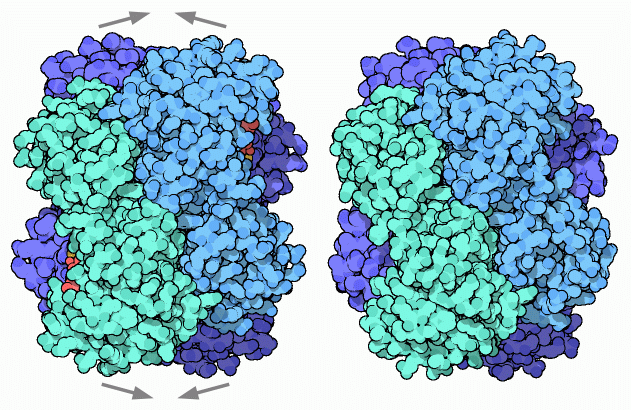
Phosphofructokinase
A hint: when you are looking at the structures of phosphofructokinase in the PDB, be sure to download the entire biological assembly, which contains four subunits!
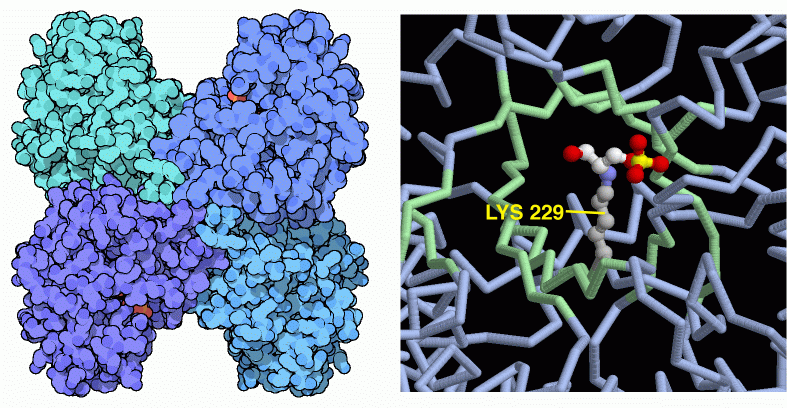
Fructose 1,6-bisphosphate Aldolase
The aldolase enzyme used by most bacteria is different than the aldolases that we have in our cells. It uses two metal ions instead of a special lysine amino acid. You can look at an example of a bacterial aldolase in PDB entry 1zen . Be sure to look for the metal ions! Also, take a look at the unusual enzyme made by hot-spring archaebacteria, in PDB entry 1ojx . It uses a lysine in the active site, like our enzyme, but is composed of ten chains in a huge molecular complex.
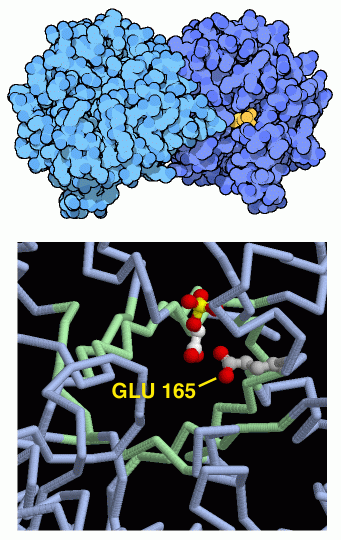
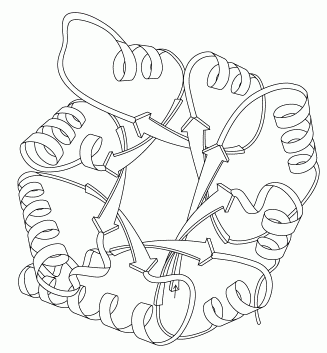
Triose Phosphate Isomerase
When you are looking at this structure yourself, also notice that the active site is in the middle of a "beta barrel:" a cylindrical arrangement of extended strands, all colored green in the close-up picture here. The cartoon picture, drawn by Jane Richardson, shows the folding of the chain in the protein, with an inner ring of extended beta strands surrounded by an outer ring of alpha helices. Notice how each of the helices connects two neighboring strands in the barrel. As you are exploring the structures of the ten glycolytic enzymes, notice that several other enzymes are also constructed with this beautiful folding pattern.
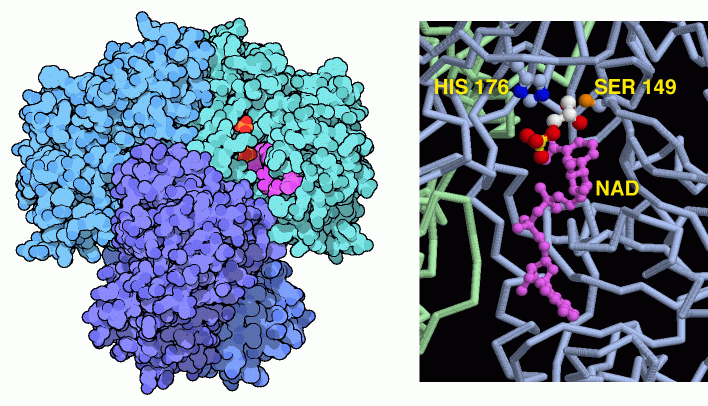
Glyceraldehyde-3-Phosphate Dehydrogenase
Glyceraldehyde-3-phosphate dehydrogenase is composed of four identical subunits. Many of the structures of this enzyme in the PDB, such as the human form shown here on the left from PDB entry 3gpd , have NAD bound in all four active sites, as well as two phosphate or sulfate ions. One ion is bound in the site occupied by the phosphate group in the sugar molecule, and the other is thought to correspond to the site that positions the incoming phosphate ion for the reaction. PDB entry 1nqo captures the first step in the reaction, when the substrate molecule binds. A nearby cysteine amino acid will then attack the molecule, forming a bond with one carbon atom. The bond is then broken when the phosphate is attached. In this structure, the cysteine is changed to a less active serine to allow study. A nearby histidine also assists in the reaction.
When you are looking at the 1nqo structure, be sure to download the proper biological unit. The primary PDB file contains four chains, but they are not the proper biological tetramer.
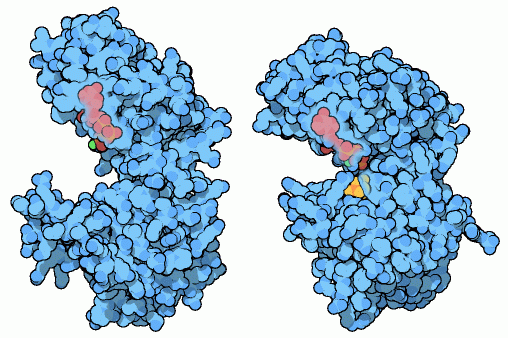
Phosphoglycerate Kinase
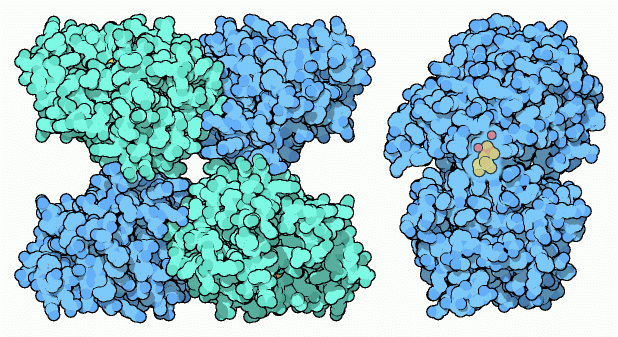
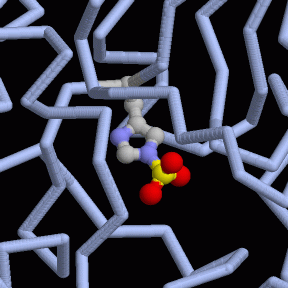
Phosphoglycerate Mutase
The enzyme in our cells uses a special histidine amino acid, like the one shown here from a bacterial enzyme in PDB entry 1e58 . This histidine extracts the phosphate and places it back in a different place. Actually, the enzyme does the reverse of this: it first places a phosphate on the molecule, so that two are attached, then it takes off the other one. In order to do this, the enzyme must be charged with this extra phosphate molecule before it can perform its reaction. A small intermediate molecule--2,3- bisphosphoglycerate--delivers these reactive phosphates to the enzyme. After the enzyme is charged, it remains active for a minute or two (busily performing its reaction many times) before the phosphate falls off and has to be replaced.
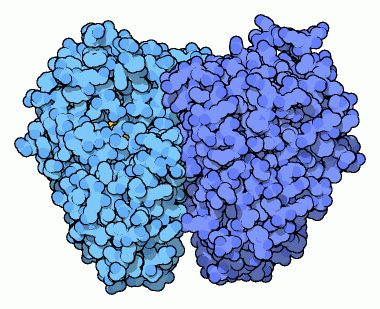
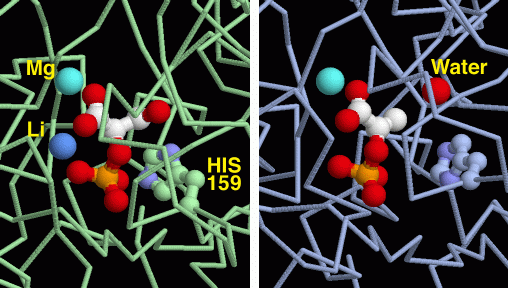
Enolase
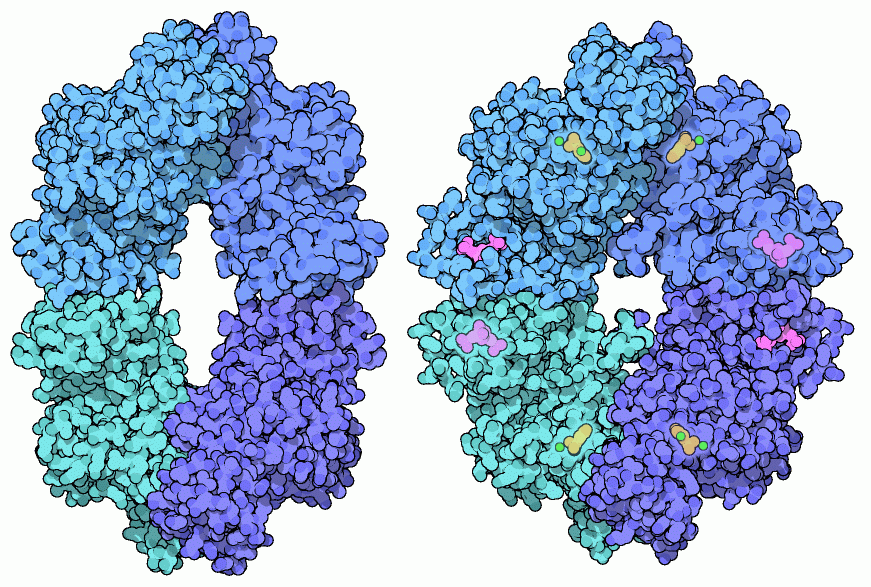
Pyruvate Kinase
The exit gate of glycolysis is guarded by a pyruvate kinase, ensuring that ATP is made only when needed. Like hemoglobin, it is progressively activated as the levels of its starting materials rise. It is also activated by the presence of phosphorylated sugars, which indicate that plenty of raw materials are available. Conversely, it is inhibited by molecules that are plentiful when the cell has enough energy, such as ATP and amino acids. Pyruvate kinase is an allosteric enzyme that senses the levels of all of these molecules and changes shape based on the result. It is composed of four flexible subunits arranged in a diamond shape. The whole complex flexes as different molecules bind. The active site is shown near the top and bottom here, and a separate regulatory site is more towards the center. The bacterial structure shown on the left, from PDB entry 1e0u , is in the inactive state. The yeast structure on the right, from PDB entry 1a3w , has a molecule bound in the regulatory site, shown in magenta, and has flexed into the active shape. The active site contains two metal ions, a potassium ion and a manganese ion shown here in green, that assist with the reaction.
Exploring the Structure
Hexokinase Action
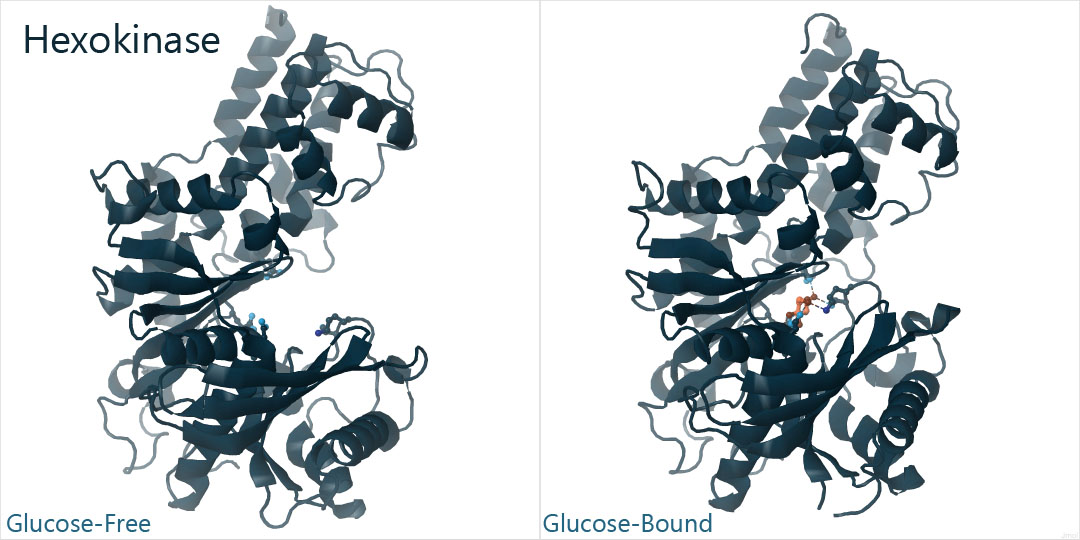
Many of the glycolytic enzymes are allosteric enzymes, meaning they change their shape when binding to their ligand. Hexokinase, the enzyme that starts glycolysis, is no exception. Structure 1ig8 shows how hexokinase is open in the absence of its substrate, leaving its binding pocket exposed. Conversely, structure 3b8a demonstrates how hexokinase closes around its bound substrate.
Select the JSmol tab to explore these structures in an interactive view.
This JSmol was designed and illustrated by Ryan Nini.
Related PDB-101 Resources
- Browse Biological Energy
- Browse Enzymes
- Browse Peak Performance
February 2004, David Goodsell
http://doi.org/10.2210/rcsb_pdb/mom_2004_2