Molecule of the Month: Green Fluorescent Protein (GFP)
A tiny fluorescent protein from jellyfish has revolutionized cell biology
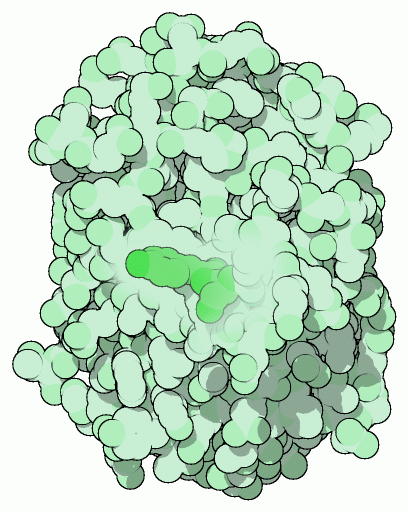
So What?
Ready-Made
Engineering GFP
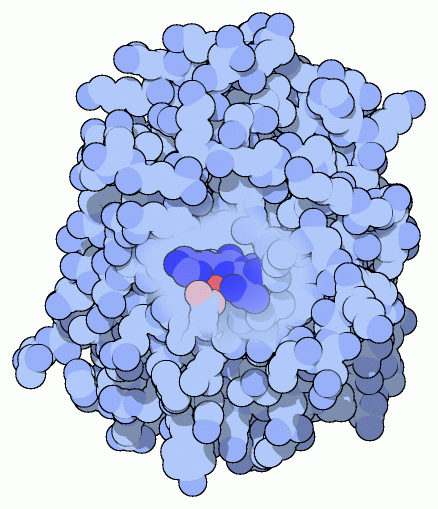
Improving GFP
Exploring the Structure
Green Fluorescent Protein
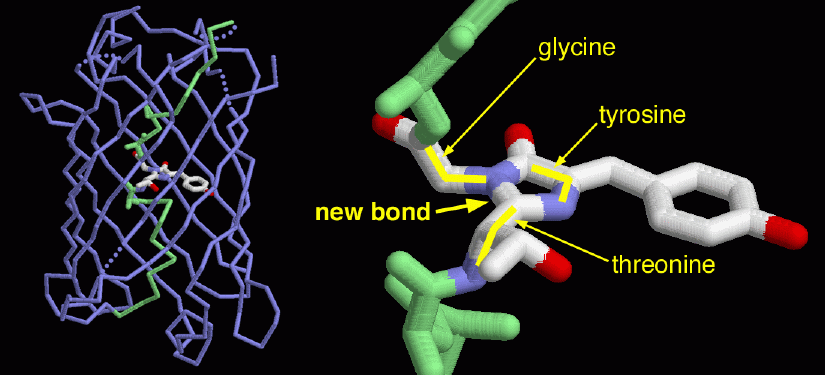
You can take a close look at the chromophore of GFP in the PDB entry 1ema . The backbone of the entire protein is shown here on the left. The protein chain forms a cylindrical can (shown in blue), with one portion of the strand threading straight through the middle (shown in green). The chromophore is found right in the middle of the can, totally shielded from the surrounding environment. This shielding is essential for the fluorescence. The jostling water molecules would normally rob the chromophore of its energy once it absorbs a photon. But inside the protein, it is protected, releasing the energy instead as a slightly less energetic photon of light. The chromophore (shown in the close-up on the right) forms spontaneously from three amino acids in the protein chain: a glycine, a tyrosine and a threonine (or serine). Notice how the glycine and the threonine have formed a new bond, creating an unusual five-membered ring. To explore this structure in more detail, click on the image for an interactive JSmol.
Topics for Further Discussion
Related PDB-101 Resources
- Browse Biotechnology
- Browse Nanotechnology
- Browse Biological Energy
- Browse Biology of Plants
- Browse Bioluminescence and Fluorescence
References
- 1kys: D.P. Barondeau, C.J. Kassmann, J.A. Tainer & E.D. Getzoff (2002) Structural chemistry of a green fluorescent protein Zn biosensor. Journal of the American Chemical Society 124, 3522-3524.
- R.Y. Tsien (1998) The green fluorescent protein. Annual Review of Biochemistry 67, 509-544.
- 1ema: M. Ormo, A.B. Cubitt, K. Kallio, L.A. Gross, R.Y. Tsien & S.J. Remington. Crystal structure of the Aequorea victoria green fluorescent protein. Science 273, 1392-1395.
- 1gfl: F. Yang, L.G. Moss & G.N. Phillips (1996) The molecular structure of green fluorescent proteins. Nature Biotechnology 14, 1246-1251.
June 2003, David Goodsell
http://doi.org/10.2210/rcsb_pdb/mom_2003_6